The goal of our project is to measure picometer level displacements and nanoradian tilts over time scales of thousand seconds, equivalent to mHz frequencies, with compact and scalable interferometry. Within geo-Q we have been following a novel approach, so-called deep frequency modulation interferometry (DFMI) [1,2] for the development of suitable interferometers. DFM combines strong laser frequency modulations with intentional unequal arm length interferometers, creating effectively strong phase modulations in the interferometer outputs. By applying a fit algorithm, the interferometric phase can be extracted from the resulting interferogram in real-time.
First experimental tests of this technique have shown promising results with table-top optical set-ups [3]. Our measurements indicate that DFMI has the potential to achieve performance levels comparable to the heterodyne interferometry that has been used in the LISA pathfinder mission and is going to be used in LISA (for information on both missions, please visit lisamission.org)
To investigate the critical limits of DFM in terms of noise, dynamic range and frequency reference stability we are implementing quasi-monolithic ultra-stable interferometers for in-vacuum operation to reduce the influence of environmental noise. A Mach-Zehnder interferometer was implemented and tested for its frequency stability, achieving 40fm/sqrt(Hz) noise levels at 1Hz using a simple balanced DC readout scheme [4], demonstrating such an interferometer as a viable alternative candidate for the LISA frequency stabilization, which is done via a reference cavity.
A photograph showing the construction of the Mach-Zehnder interferometer in one of our clean rooms is shown in figure 01.
Currently we are prototyping compact optical heads capable of probing from two sides the motion of a test mass, in our case a gold-coated mirror. By actuating on the mirror position and tilt we can then test the displacement performance of DFMI in a realistic test-bed. Tuning the dynamic range of the motion and investigating the effects due to amplitude noise, non-linear laser frequency modulation, and stray light, this experiment will enable us to perform noise hunting, opening the path to revealing the final performance of DFMI.
Besides the optical design, the second focus of our project is the development of a dedicated DFMI phasemeter, a high-performance measurement system capable to capture the interferometric signal. While precision is an inherent requirement for our metrology application, we also aim for real-time phase extraction of multiple channels and an active control of the laser modulation. The approach we follow in this project relies on spectral analysis and least-square-fitting.
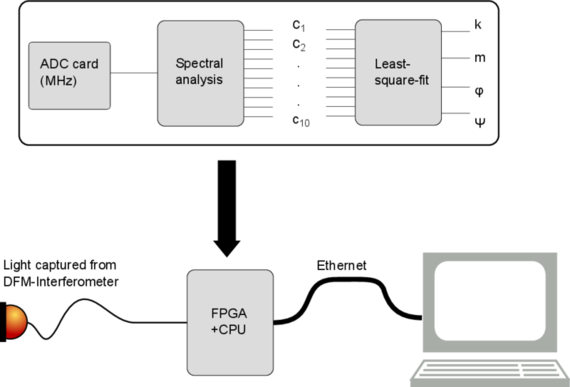
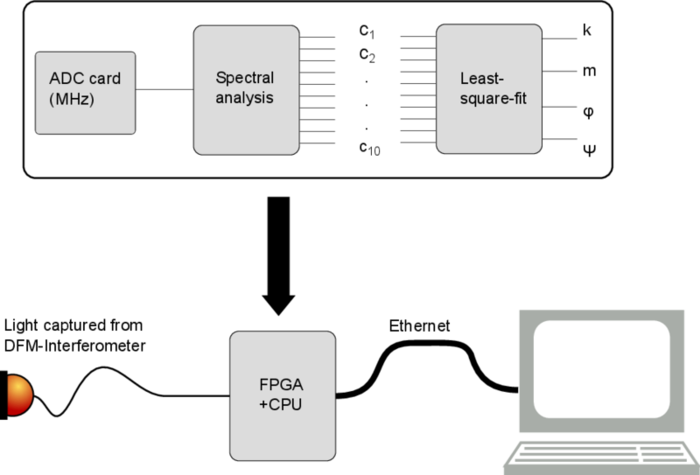
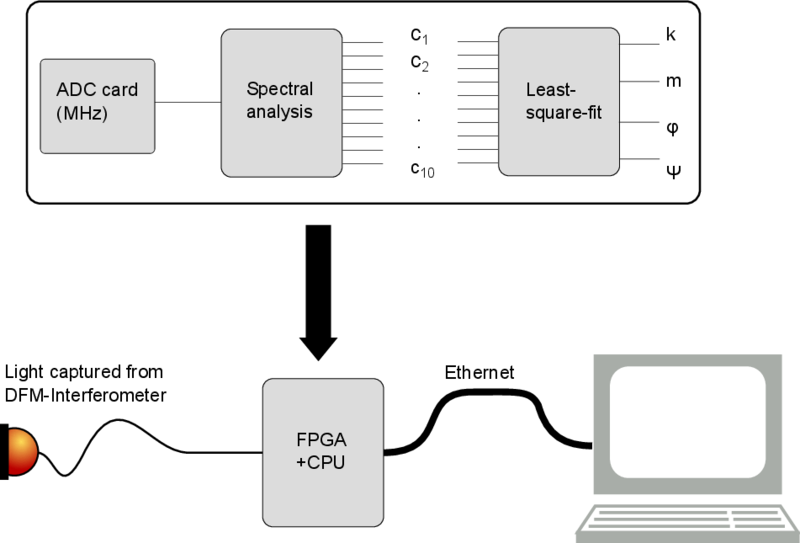
Figure 02: Schematic of the phase extraction algorithm and the hardware used: the interferometer time-series is sampled via an ADC card and a spectral analysis is performed to obtain harmonic amplitudes c_n. Those are used by a least-square-fit to obtain the interferometric parameters, in particular the interferometer phase phi. In the current setup, all these parts are implemented on a single SoC (System-on-Chip).
For its implementation, we use modern hardware in form of an SoC (system-on-chip). These very flexible and compact devices can be described as a combination of Microprocessor and field programmable gate array (FPGA). They allow for the programming of software as well as customized digital parallel circuits in just one single chip. In our case, the digital circuits were programmed to implement the spectral analysis while the processor runs software performing the least-square-fit. Using such a system, we were able to successfully track the motion of a mirror in a DFMI setup. While our approach has proved to be robust, other methods are in development and still allow for creative work. In the best case, one of them can surpass the current benchmark method in term of compactness or precision.
[1] Gerberding O. (2015): Deep frequency modulation interferometry, Opt. Express 23, 14753-14762 (2015) DOI: 10.1364/OE.23.014753
[2] Isleif K.-S., Gerberding O., Mehmet M., Schwarze T.S., Heinzel G. and Danzmann K. (2016): Comparing interferometry techniques for multi-degree of freedom test mass readout, Journal of Physics: Conference Series 716, 012008 (2016) DOI: 10.1088/1742-6596/716/1/012008
[3] Isleif K.-S., Gerberding O., Schwarze T.S., Mehmet M., Heinzel G. and Guzmán Cervantes F. (2016): Experimental demonstration of deep frequency modulation interferometry, Opt. Express 24, 1676-1684 (2016) DOI: 10.1364/OE.24.001676
[4] O.Gerberding, K.-S. Isleif, M. Mehmet, K. Danzmann and G Heinzel (2017): Laser-Frequency Stabilization via a Quasimonolithic Mach-Zehnder Interferometer with Arms of Unequal Length and Balanced dc Readout, Phys. Rev. Applied 7, 024027 (2017) http://journals.aps.org/prapplied/abstract/10.1103/PhysRevApplied.7.024027
Principal Investigators
Callinstr. 38
30167 Hannover
Callinstr. 38
30167 Hannover
Callinstr. 38
30167 Hannover
Callinstr. 38
30167 Hannover